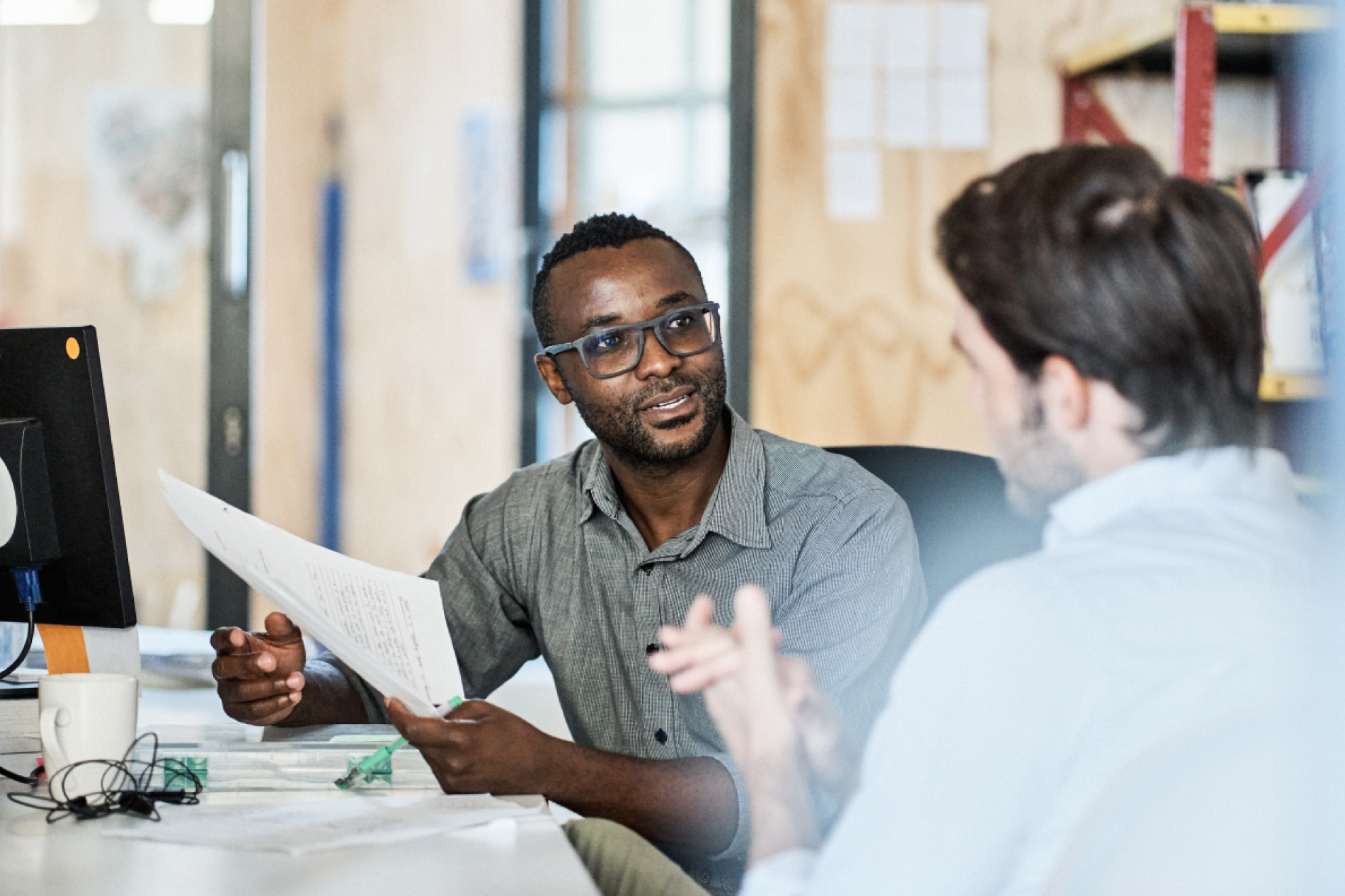
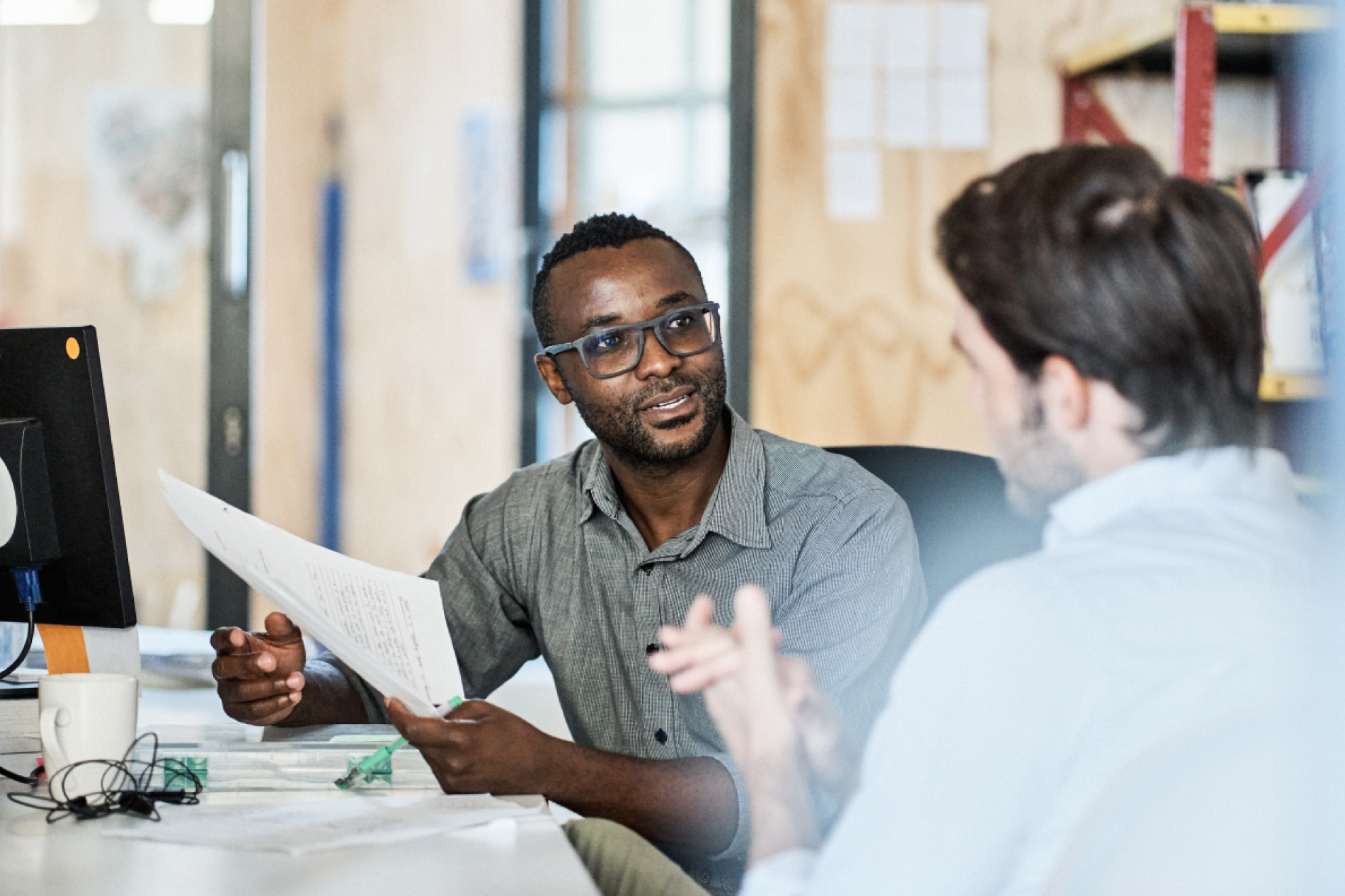
By Michael Kanellos, Head of Influencer Relations, Marvell
Computer architects have touted the performance and efficiency gains that can be achieved by replacing copper interconnects with optical technology in servers and processors for decades1.
With AI, it’s finally happening.
Marvell earlier this month announced that it will integrate co-packaged optics (CPO) technology into custom AI accelerators to improve the bandwidth, performance and efficiency of the chips powering AI training clusters and inference servers and opening the door to higher-performing scale-up servers.
The foundation of the offering is the Marvell 6.4Tbps 3D SiPho Engine announced in December 2023 and first demonstrated at OFC in March 2024. The 3D SiPho Engine effectively combines hundreds of components—drivers, transimpedance amplifiers, modulators, etc.—into a chiplet that itself becomes part of the XPU.
With CPO, XPUs will connect directly into an optical scale-up network, transmitting data further, faster, and with less energy per bit. LightCounting estimates that shipments of CPO-enabled ports in servers and other equipment will rise from a nominal number of shipments per year today to over 18 million by 20292.
Additionally, the bandwidth provided by CPO lets system architects think big. Instead of populating data centers with conventional servers containing four or eight XPUs, clouds can shift to systems sporting hundreds or even thousands of CPO-enhanced XPUs spread over multiple racks based around novel architectures—innovative meshes, torus networks—that can slash cost, latency and power. If supercomputers became clusters of standard servers in the 2000s, AI is shifting the pendulum back and turning servers into supercomputers again.
“It enables a huge diversity of parallelism schemes that were not possible with a smaller scale-up network domain,” wrote Dylan Patel of SemiAnalysis in a December article.
By Michael Kanellos, Head of Influencer Relations, Marvell
What happened in semis and accelerated infrastructure in 2024? Here is the recap:
1. Custom Controls the Future
Until relatively recently, computing performance was achieved by increasing transistor density à la Moore’s Law. In the future, it will be achieved through innovative design, and many of those innovative design ideas will come to market first—and mostly— through custom processors tailored to use cases, software environments and performance goals thanks to a convergence of unusual and unstoppable forces1 that quietly began years ago.
FB NIC on display at OFC
By Michael Kanellos, Head of Influencer Relations, Marvell
With AI computing and cloud data centers requiring unprecedented levels of performance and power, Marvell is leading the way with transformative optical interconnect solutions for accelerated infrastructure to meet the rising demand for network bandwidth.
At the ECOC 2024 Exhibition Industry Awards event, Marvell received the Most Innovative Pluggable Transceiver/Co-Packaged Module Award for the Marvell® COLORZ® 800 family. Launched in 2020 for ECOC’s 25th anniversary, the ECOC Exhibition Industry Awards spotlight innovation in optical communications, transport, and photonic technologies. This recognition highlights the company’s innovations in ZR/ZR+ technology for accelerated infrastructure and demonstrates its critical role in driving cloud and AI workloads.
By Michael Kanellos, Head of Influencer Relations, Marvell
Coherent optical digital signal processors (DSPs) are the long-haul truckers of the communications world. The chips are essential ingredients in the 600+ subsea Internet cables that crisscross the oceans (see map here) and the extended geographic links weaving together telecommunications networks and clouds.
One of the most critical trends for long-distancer communications has been the shift from large, rack-scale transport equipment boxes running on embedded DSPs often from the same vendor to pluggable modules based on standardized form factors running DSPs from silicon suppliers tuned to the power limits of modules.
With the advent of 800G ZR/ZR+ modules, the market arrives at another turning point. Here’s what you need to know.
It’s the Magic of Modularity
PCs, smartphones, solar panels and other technologies that experienced rapid adoption had one thing in common: general agreement on the key ingredients. By building products around select components, accepted standards and modular form factors, an ecosystem of suppliers sprouted. And for customers that meant fewer shortages, lower prices and accelerated innovation.
The same holds true of pluggable coherent modules. 100 Gbps coherent modules based on the ZR specification debuted in 2017. The modules could deliver data approximately 80 kilometers and consumed approximately 4.5 watts per 100G of data delivered. Microsoft became an early adopter and used the modules to build a mesh of metro data centers1.
Flash forward to 2020. Power per 100G dropped to 4W and distance exploded: 120k connections became possible with modules based on the ZR standard and 400k with the ZR+ standard. (An organization called OIF maintains the ZR standard. ZR+ is controlled by OpenROADM. Module makers often make both varieties. The main difference between the two is the amplifier: the DSPs, number of channels and form factors are the same.) ®
The market responded. 400ZR/ZR+ became adopted more rapidly than any other technology in optical history, according to Cignal AI principal analyst Scott Wilkinson.
“It opened the floodgates to what you could do with coherent technology if you put it in the right form factor,” he said during a recent webinar.
This article is part four in a series on talks delivered at Accelerated Infrastructure for the AI Era, a one-day symposium held by Marvell in April 2024.
Silicon photonics—the technology of manufacturing the hundreds of components required for optical communications with CMOS processes—has been employed to produce coherent optical modules for metro and long-distance communications for years. The increasing bandwidth demands brought on by AI are now opening the door for silicon photonics to come inside data centers to enhance their economics and capabilities.
What’s inside an optical module?
As the previous posts in this series noted, critical semiconductors like digital signal processors (DSPs), transimpedance amplifiers (TIAs) and drivers for producing optical modules have steadily improved in terms of performance and efficiency with each new generation of chips thanks to Moore’s Law and other factors.
The same is not true for optics. Modulators, multiplexers, lenses, waveguides and other devices for managing light impulses have historically been delivered as discrete components.
“Optics pretty much uses piece parts,” said Loi Nguyen, executive vice president and general manager of cloud optics at Marvell. “It is very hard to scale.”
Lasers have been particularly challenging with module developers forced to choose between a wide variety of technologies. Electro-absorption-modulated (EML) lasers are currently the only commercially viable option capable of meeting the 200G per second speed necessary to support AI models. Often used for longer links, EML is the laser of choice for 1.6T optical modules. Not only is fab capacity for EML lasers constrained, but they are also incredibly expensive. Together, these factors make it difficult to scale at the rate needed for AI.